Dr. Gerald S. Hecht
Assistant
Professor
of Psychology
College
of Sciences
webmaster@psiwebsubr.org
PSYC
381 - Sensation & Perception Exam 3 Study Guide
Vision
Part II: From the Eye to the Brain
THE
IMAGE DRAWN onto the retina by eye optics is
transduced
chemically in the rods and cones. This chemistry closes channels in
their plasma membranes, and that increases their polarity. Their
synapses onto bipolar cells slow or stop in their activity, and this releases
bipolars from inhibition. Both of these cells are also leaky
(pacemakers), and they can activate their synapses--which are also
inhibitory.
The principal
target of bipolars is ganglion cells, which have been pacing action
potentials to higher brain centers in a disordered fashion. The
activation of bipolars because appropriate light has struck their
receptor cells--imposes inhibitory order onto this ganglion cell
discharge, creating a rough image that is projected centrally. That
image will be "fuzzy" in the case of rods because information from
entire "gangs" of them communicate with a single bipolar cell--cones
however produce crisp, clean sharp images stemming from their "direct
line" to the bipolars (each cone gets to have "its own" bipolar cell).
Do I have to draw you a picture?
Ok, here are two pictures--one of the rod situation and another of the
cone situation:
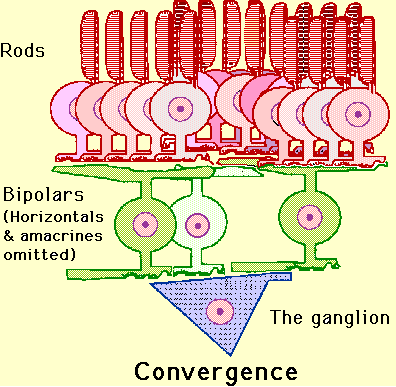
This is the
data of the optic nerve. And a nice summary (I think) of the material
covered on the last exam.
Optic Tract and Chiasm
GRANULE
CELL AXONS converge from the entire retina on an area medial to the
visual axis of each eye, and at this point they gain their myelin
sheath
(which would not have been transparent on the surface of the retina!)
and exit the retina and the eyeball as the optic "nerve." In humans
there are about 1,000,000 axons in each nerve, and these take up space.
Which means that where they exit the eye, there is no room for retinal
columns, and this creates a blind spot. Since the two spots in the two
eyes do not coincide in their retinal fields, one eye sees what the
other does not. You learn to ignore this hole in your
vision. The nerve passes through a tendonous ring,
which is the origin of the recti muscles, and enters the cranial cavity
via the orbital foramen. At this point the tough fibrous sheath of the
nerve (which is continuous with the sclera) merges with the dura mater
lining the cranial cavity. Beyond this point, the CNS affiliation of
the
tract is evident from the various meningeal relations (figure: "Optic
Tract & Meninges). In its route, the tracts are still passing
medially as well as posteriorly,
and the nerves of the two sides meet at the optic
chiasm.
In vertebrates
with laterally-directed eyes, the nerves may cross entirely at this
decussation, but in man, where the eyes have converged with much
binocular overlap, only about half of these axons cross over. These
visual fields were shown in detail in the description of retinal
function (The last study guide). The effect of this mixed projection to
the optic cortex is that the entire visual field (as opposed to retinal
field) is projected to the contralateral (opposite side) cortex. If you
observe carefully, you may see this. Often when you first awake, your
eyes will have moved out of coherence, and when you first open them,
one
of the images will shift to match the other slowly enough for you to
see
it "snap" into place.
Lateral Geniculate
Body
The
optic
tract (as it is now called) enters the diencephalon
and
extends to the lateral geniculate nucleus
("G" on the image pathway drawing above).
The lateral geniculate nucleus
(LGN) of the thalamus is a part of the brain, which is the primary
processor of visual information, received from the retina, in the CNS.
The LGN receives information directly from the retina, and sends
projections directly to the primary visual cortex. In addition, it
receives many strong feedback connections from the primary visual
cortex.
Ganglion cells of the retina send axons to the LGN through the optic
nerve. Although it is generally considered to be a cranial nerve, and
is always listed as cranial nerve II, in reality the retina and optic
nerve arise as an outpocketing of the developing diencephalon. Rather
than a proper nerve, then, the optic nerve is really a tract of the
brain.
The LGN is a distinctively layered structure ("geniculate" means "bent
like a knee"). In most primates, including humans, it has six layers of
cell bodies with layers of neuropil in between, in an arrangement
something like a club sandwich or layer cake, with cell bodies of LGN
neurons as the "cake" and neuropil as the "icing".
These six layers contain two types of cells. The cells in layers 1 and
2 are large, or magnocellular ; others in layers 3, 4, 5, and 6 are
smaller, or parvocellular. (The Latin prefix "parvo-" means "small";
some authors prefer the term parvicellular. If you're searching for
more information, try both spellings.)
Between each of the M and P layers lies a zone of very small cells: the
interlaminar, or koniocellular (K), layers. K cells are functionally
and neurochemically distinct from M and P cells and provide a third
channel to the visual cortex.
The magnocellular, parvocellular, and koniocellular layers of the LGN
correspond with the similarly-named types of ganglion cells.
M and P Cells
Magnocellular cells (commonly called M cells) have large cell bodies,
use a relatively short time to process information, and are part of a
visual processing system that tells the brain where something is. This
system operates quickly but without much detail. They are found in
layers 1 and 2 of the LGN, those layers more ventrally located which
are next to the incoming optic tract fibers.
Parvocellular cells (commonly called P cells) have small cell bodies,
use a relatively long time to process information, and are part of a
visual processing system that tells the brain what something is. This
system operates more slowly and with lots of information about details.
For example, these cells carry color information while magnocellular
cells do not. Parvocellular cells are found in layers 3, 4, 5 and 6.
Ipsilateral and Contralateral Layers
Additionally, the layers are divided up so that the eye on the same
side (the ipsilateral eye) sends information to layers 2, 3 and 5 while
the eye on the opposite side (the contralateral eye) sends information
to layers 1, 4 and 6. (A simple mnemonic for this is that 2 + 3 = 5
while 1 + 4 does not equal 6, so it is "contra"ry to your knowledge of
math.)
Remember that in visual perception, the right eye gets information from
the right side of the world (the right visual field) as well as the
left side of the world (the left visual field). You can confirm this by
covering your left eye: the right eye still sees to your left and
right, but on the left side, your vision is partially blocked by your
nose.
In the LGN, the corresponding information from the right and left eyes
is "stacked" so that a toothpick driven through the club sandwich of
layers 1 through 6 would hit the same point in visual space six
different times.
Retinotopic
Map
The spatial position of the
ganglion cells within the retina is preserved by the spatial
organisation of the neurons within the LGN layers. The posterior LGN
contains neurons whose receptive field are near the fovea. Progressing
from posterior to anterior, the receptive field locations become
increasingly peripheral in the retina (see Erwin et al., 1999). This
spatial layout is called retinotopic organization because the
topological organization of the receptive fields in the LGN parallels
the organization of the retina.
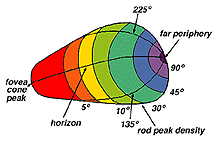
LGN Output
Information leaving the LGN travels out on the optic radiations, which
form part of the retrolenticular limb of the internal capsule.
The axons which leave the LGN go to V1 visual cortex (areas 17 & 18)and generally end
in layer IV.
Axons from layer VI of visual cortex send information back to the
LGN.
Primary Visual Cortex
POSTSYNAPTIC
FIBERS after the lateral geniculate body fan out in the optic
radiation, a tract that extends back to the occipital lobe of the
cerebral hemisphere. The primary visual cortex includes Brodmann areas
17, 18, and 19. Appreciation of the image is done in a variety of
association areas, particularly on the parietal and temporal lobes of
the hemisphere.
Area 17
Area 17
essentially draws the lines and boundaries of objects in the image.
Lateral geniculate output is directed to this cortex. This neuronal
output is translated into simple and complex
fields of cortical columns.
Points
of light, which can stimulate the retina very effectively, cause almost
no response in visual cortex. Instead, lines of patterns representing
the borders between parts of the retina responding to the distal
stimulus (light) and neighboring parts of the retina that are not cause
a strong contrast reaction within rectangular fields of cortical
columns. This is the basis of our ability to see edges of objects and
the boundaries between objects in the world.
Cortical
fields are usually depicted as a central rectangle flanked by two
surround rectangles. The placement of excitation within the boundaries
of these three rectangles is based on the projections from the
retina/geniculate, but an expanded rule is applying--multiple centers
in
line are drawing the image. With simple
cells, an image aligned along a row of appropriate centers yields a
very
strong response. Moving that line into the surround or changing the
orientation (angle) of the line has a strong effect on signal; if the
bar is rotated 90° into a horizontal position, the signal generated by
these particular cortical fields would disappear entirely at about a
45°
angle.
Complex fields
are generated in a different layer of the cortex from the simple
fields.
Complex fields are usually larger in perimeter than are simple fields.
On/off relationships are not so well demarked, Angle of the the
stimulus
is not so important, but movement of a wave of excitation across a
field
(representing movement of the image across the retinal ganglion cells)
has a very strong effect.
The cortex of
area 17 is a mixture of simple and complex fields, and their
interaction
draws both the outlines (including stopping points) and relative
movement of visual images. Apparently in the hierarchy of evaluation,
the simple fields analyze multiple cells of the eye/geniculate input,
while complex fields analyze multiple simple fields.
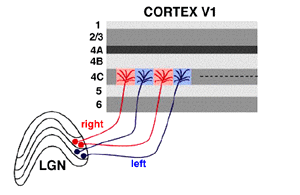
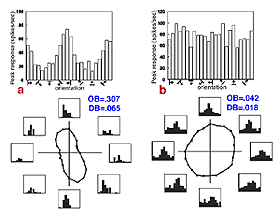
Area 18
Area
18 participates in the coloration of the drawn image
of
area 17, but 17 is involved with this process as well. The actual
process of recognition of colors is only poorly understood and involves
layers of the cortex which are organized as "blobs" outside the system
of simple and complex fields. Since an on-response might be related to
a
green cone, while off-response cells may be cones of another color or
also of green--it gets very complex! The eye is also able to correct
for
variation of ambient light color (such as, for example, sunset) in
reconstructing the color shades of objects.
Area 19
Area 19 of the occipital
cortex is a motor association area which receives input from the
lateral
geniculate and many other regions. This area is aware in a geographical
sense, translating the image into motor coordinates that are referred
onward to the mesencephalic tectum, described above. These motor
calculations track the movement of objects and also changes in position
of the eyes and of the head so that the image is not blurred and is
projected to the "correct" position in the outside world. But area 19
doesn't actually "see" anything.
Retina to Visual
Cortex
Blindsight: Remember Area
19 of the visual cortex doesn't actually "see", but rather
directs
motor movements around detection of motion in subcortical parts of the
visual system. There are numerous clinical cases primarily involving
head injuries and strokes in which Area 17 and associated
pathways are destroyed or severely damaged, but Area 19 and its
pathways
are still intact. This results in a fascinating type of blindness
known as "blindsight". If you held a baseball up in front of
someone with this syndrome they would respond as any blind person
would... they would be unable to tell you the name of the object you
are
holding (in fact they wouldn't even know that you were holding an
object-- they are blind after all). HOWEVER.... if you proceed to toss
the baseball to them...they will, almost as if by magic, raise their
arm
and catch the baseball!! If you then ask them to look at the object in
their hand and describe its appearance.... they won't be able to! They
are now "blind again"
... thus there are really TWO
VISUAL PATHWAYS in the brain:
1. PRIMARY VISUAL PATHWAY
The pathway that leads
to the conscious awareness of objects in the world (our "normal" sense
of vision). These pathways lead from LGN
to Area 17 and Area
18 in the cortex of the brain.
2. SECONDARY VISUAL PATHWAY
A second visual pathway
which allows are body to reflexively respond to moving objects (without
our even having to think about it or be aware of it) such as baseballs
flying towards our face! These pathways lead from LGN to Superior Colliculus to Area 19
in
the cortex of the brain.